- 易迪拓培训,专注于微波、射频、天线设计工程师的培养
在便携应用中设计 D 类音频放大器(英)
Integration of audio into portable devices such as cellphones, DVD players, laptop computer and gaming devices has increased to the point where designers are faced with squeezing more and more features like MP3 and streaming video into these end-equipments, yet at the same time, maintaining or even reducing the overall power budget.
This has led to the adoption of many new product technologies including Class-D audio power amplifiers (APAs). These amplifiers allow designers to save battery life because Class-D amplifiers are more efficient than traditional Class-AB or linear amplifiers. To maximize the added benefits of using Class-D amplifiers it is important to understand the fundamental difference in operation between Class-D and Class-AB amplifiers, since each amplifier technology requires different considerations when designing systems that incorporate audio. The difference in operation between both technologies will be explained in this document.
A discussion of design considerations will follow the section on Class-D operation. The importance of layout will be discussed when using Class-D amplifiers and how to select and place the appropriate external components on a board. EMI results that where taken with good board layout and component selection are included in this section.
Finally, how to measure fundamental parameters, such as output power and THD+N of a Class-D amplifier are explained in detail.
Efficiency of Class-D Amplifiers vs. Class-AB
Class-D amplifiers offer much better efficiency than Class-AB amplifiers. The higher the efficiency of the amplifier the less power is wasted as heat from the battery. Figure 1 shows a power dissipation versus output power curve for the newest Class-D stereo audio power amplifier, TPA2012D2, compared to the theoretical power dissipation of a traditional linear amplifier. This graph shows how the power dissipation varies with output power for both types of amplifier technologies. The improved efficiency of Class-D amplifiers delivers two advantages to audio designers. For portable, battery-operated applications, the battery will last longer since less power is wasted by the amplifier as heat. Furthermore, since the amplifier dissipates less heat, thermal problems are reduced compared to a Class-AB device.
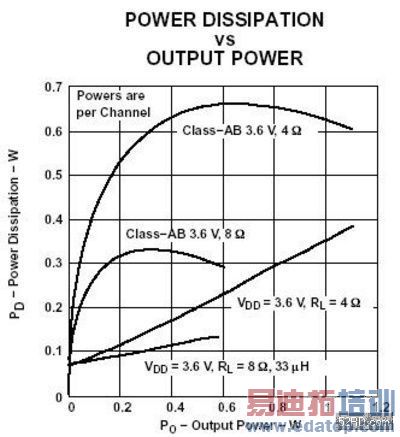
Figure 1: Power dissipated versus output power for the TPA2012D2 compared to the theoretical power dissipation of a Class-AB amplifier.
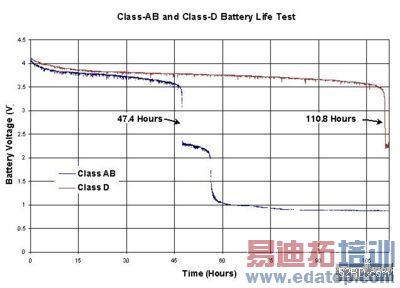
Figure 2: Battery discharge time for a Class-D vs. Class-AB audio power amplifier.
As can be seen from the graph, the Class-AB amplifier (red line) drains the battery much faster than the Class-D amplifier (blue line). Class-AB amplifiers dissipate more power than Class-D for the same output power and which reduces the battery usage time.
Efficiency of Class-AB Amplifiers
So, why are Class-AB amplifiers much less efficient than Class-D?
A Class-AB (linear) amplifier consumes a fixed amount of supply current for the desired output voltage. The supply current equals the output current in a bridge-tied-load (BTL) Class-AB amplifier plus the quiescent current. Figure 3 illustrates the currents in a simplified Class-AB, H-bridge output stage.
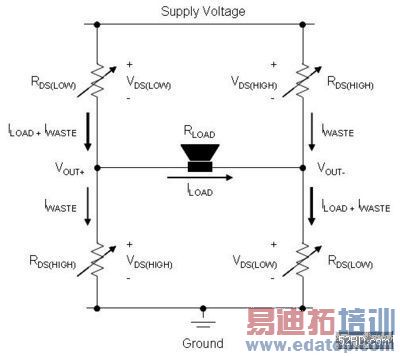
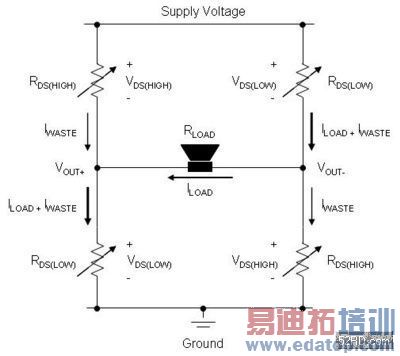
Figure 3: Simplified H-bridge for a Class-AB amplifier.
The output MOSFETs are drawn as variable resistors, whose resistance changes as a function of amplifier output voltage. These MOSFETs vary their drain-source resistance as the output voltage changes. The current flowing from the supply through the load causes a voltage drop across all the MOSFETs. These currents multiplied by the voltage drop across the MOSFETs create the large power dissipation in the amplifier. This power dissipation in these MOSFETs is the reason Class-AB amplifiers are inefficient when compared to Class-D amplifiers.
Efficiency of Class-D Amplifiers
So, why do Class-D amplifiers dissipate less power?
A Class-D audio amplifier, in contrast to a Class-AB audio amplifier, delivers a fixed amount of power into the load for a given period. The Class-D amplifier creates a PWM signal that makes the output voltage switch between the supply rails, yielding very little voltage drop across the output transistors when driving current to the load. The ideal output transistors in the H-bridge for a Class-D amplifier would have zero RDS(ON) (drain-to-source resistance when "ON") and infinite RDS(OFF) (drain-to-source resistance when "OFF"). In this case, the Class-D amplifier output stage would deliver an equal amount of power from the supply to the load (this excludes losses through bias-currents and gate-drive). Due to the fact the all MOSFETs have some RDS(ON) and a finite RDS(OFF), there is some power loss. Figure 4 shows the MOSFETs as simplified switches that are either "ON" or "OFF".
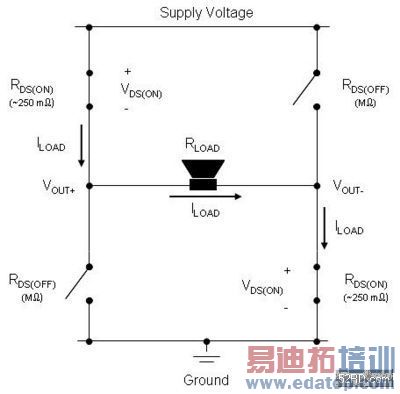
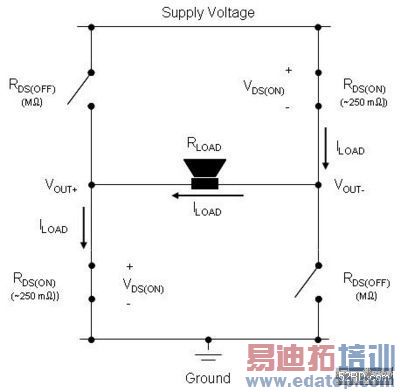
Figure4: Simplified H-bridge for a Class-D amplifier.
In Figure 4, as the current flows from the supply through the first MOSFET that is "ON", through the load and finally through the last MOSFET that is "ON". Because the two MOSFETs are fully saturated "ON", there is only a slight voltage drop across them. A voltage divider is formed by RDS(ON), RDS(OFF) and the output load or speaker, RL. The RDS(ON) of the MOSFETs is very small, so that there is virtually no voltage drop across them. In contrast, when the MOSFETs or "OFF" the value of RDS(OFF) is large, but there will be negligible current flowing through them. As a result, Class-D amplifiers are very efficient since only a small amount of power is dissipated by the output MOSFETs compared to Class-AB amplifiers.
While selection of an audio amplifier is very important to the design of your circuit, the layout of the circuit on the PC board can determine the success or failure of the circuit to operate according to specs. Some PCB considerations are power and ground routing, input and output routing, component selection and placing.
Laying out PCB boards with Class-D Amplifiers
One critical layout consideration is the input and outputs traces. The routing of these traces is important because the inputs can pick-up noise from the output and the outputs can radiate noise to the rest of the circuit. Routing the input traces is important since any noise that is coupled onto the inputs is amplified by the amplifier gain and may be heard as undesirable distortion or noise. Audio amplifiers that have fully-differential inputs have the advantage of rejecting common-mode noise on the input signals. Therefore, even if the input signal is single-ended, properly routing the inputs allows increased immunity to noise even in a very noisy environment.
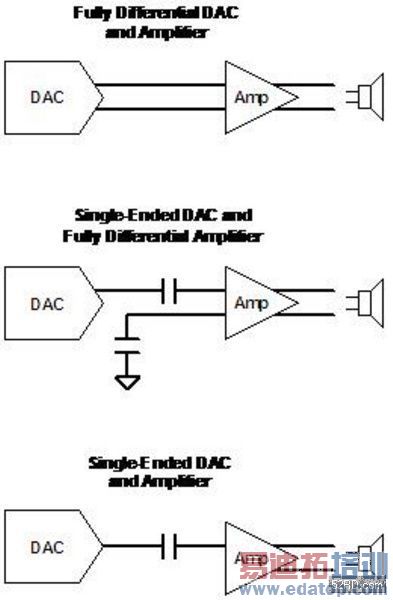
Figure 5: Different input Implementations
In a fully-differential architecture, the inputs should be routed close together and in parallel to ensure any noise coupling onto one side of the amplifier’s input will couple onto the other side with the same magnitude.
Many designs only permit a single-ended audio signal and some customers think that a fully differential input will not benefit them in this configuration. This is not the case however, because the single-ended signal can be routed in parallel to the AC ground line of the other input. The AC grounded terminal should be routed in parallel to the audio signal and AC grounded as close to the audio signal source as possible. This allows any noise to couple onto both the audio signal and ground trace to allow maximum common mode noise rejection.
The output traces of the amplifiers are another area of that must be routed carefully. For Class-D amplifiers, the output signals are switching signals and can cause EMI if proper precautions are not taken. One problem that some circuits have is that the output traces are routed over or near the audio input signals or other sensitive signals and circuitry. This causes the outputs to radiate onto this circuitry or couple onto other signals. For this reason care must be taken when routing the output traces. An example of one common routing problem takes place with flip-open cell phones.
The lower portion of the cell phone is connected and passes data and signals to the upper portion via a flex cable. This cable contains power, ground and data signals for the LCD screen. Some cell phones also place the hands-free speaker in the upper portion of the phone. This means that the audio signal must also be routed across this cable. Routing a switching signal (Class-D audio amplifier) next to digital data signal for the LCD screen has the potential to corrupt the LCD data. Keeping the signals apart to prevent the signals from coupling and using components such as ferrite beads to remove the high frequency harmonics of the switching signal fixes the LCD screen interference problem.
The ferrite bead filter must be used properly in order for it to be most effective. The ferrite bead should be placed as close to the output pin of the IC as possible. The capacitor should then be connected to the output of the ferrite bead and grounded. Make sure that the ground connection of the capacitor is away from grounds that are for sensitive analog circuitry. If it isn’t, the high frequency signal filtered off the switching signal will pass onto the ground signal and could corrupt the sensitive analog circuitry. For Class-D amplifiers, the output trace length should also be minimized to avoid radiation that may prevent the product from passing FCC and CE requirements for EMI. Proper routing, using a ferrite bead filter and minimizing the trace length are all steps that will improve the design.
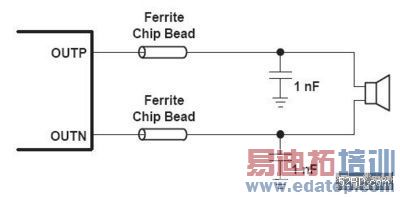
Figure 6: Typical Ferrite Bead Filter
In addition to the input and output traces, the power and ground traces are of concern since they must provide adequate power and grounding for all circuits. In routing the power and ground traces, consider where the current loops will be. Route traces such that the current flows are predictable. For the ground traces, this means using a star ground. The ground signal should be routed to a point where the power ground and analog ground will split off. These ground splits should be used for decoupling and ground for the appropriate signals. For example, Figure 7 shows decoupling for PVDD/PVCC are connected to the PGND trace and not the AGND trace. The power signal can be routed similar to the ground with a split for power and analog supplies (if required). The ground trace of the ferrite bead filter should attempt to route back to the star ground point in order to prevent the high frequency switching noise that will be present from corruption of any signals connected to the ground planes.

Figure 7: Star signal topology for power and ground for TPA2012D2 EVM